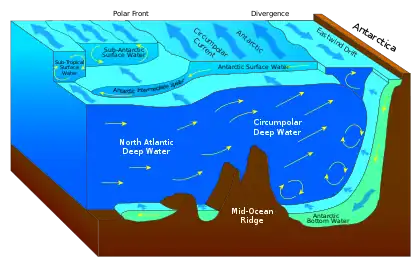
The Antarctic bottom water (AABW) is a type of water mass in the Southern Ocean surrounding Antarctica with temperatures ranging from −0.8 to 2 °C (35 °F) and absolute salinities from 34.6 to 35.0 g/kg.[1] As the densest water mass of the oceans, AABW is found to occupy the depth range below 4000 m of all ocean basins that have a connection to the Southern Ocean at that level.[2]
The major significance of Antarctic bottom water is that it is the coldest bottom water, giving it a significant influence on large-scale movement in the world's oceans through thermohaline circulation.
Initially, AABW has a high oxygen content relative to the rest of the oceans' deep waters but this depletes over time. This early oxygen abundance comes from the precursor water mass of the AABW, which is cold, relatively salty and oxygen-rich dense shelf water (DSW) formed above Antarctica’s continental shelf by wintertime cooling and brine rejection. This water sinks at four distinct regions around the margins of the continent and forms the AABW; this process leads to ventilation of the deep ocean, or abyssal ventilation.[3]
Formation and circulation
Antarctic bottom water is created in part due to the major overturning of ocean water.
Antarctic bottom water is formed in the Weddell and Ross Seas, off the Adélie Coast and by Cape Darnley from surface water cooling in polynyas and below the ice shelf.[4] A unique feature of Antarctic bottom water is the cold surface wind blowing off the Antarctic continent.[5] The surface wind creates the polynyas which opens up the water surface to more wind. This Antarctic wind is stronger during the winter months and thus the Antarctic bottom water formation is more pronounced during the Antarctic winter season. Surface water is enriched in salt from sea ice formation. Due to its increased density, it flows down the Antarctic continental margin and continues north along the bottom. It is the densest water in the free ocean, and underlies other bottom and intermediate waters throughout most of the southern hemisphere. The Weddell Sea Bottom Water is the densest component of the Antarctic bottom water.
Evidence indicates that Antarctic bottom water production through the Holocene (last 10,000 years) is not in a steady-state condition;[6] that is to say that bottom water production sites shift along the Antarctic margin over decade to century timescales as conditions for the existence of polynyas change. For example, the calving of the Mertz Glacier, which occurred on 12–13 February 2010, dramatically changed the environment for producing bottom water, reducing export by up to 23% in the region of Adelie Land.[7] Evidence from sediment cores, containing layers of cross-bedded sediments indicating phases of stronger bottom currents, collected on the Mac.Robertson shelf [8] and Adélie Land[9] suggests that they have switched "on" and "off" again as important bottom water production sites over the last several thousand years.
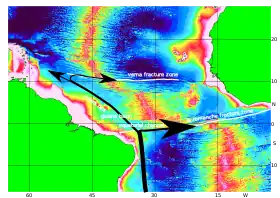
Atlantic Ocean
The Vema Channel, a deep trough in the Rio Grande Rise of the South Atlantic at 31°18′S 39°24′W / 31.3°S 39.4°W, is an important conduit for Antarctic Bottom Water and Weddell Sea Bottom Water migrating north.[10] Upon reaching the equator, about one-third of the northward flowing Antarctic bottom water enters the Guiana Basin, mainly through the southern half of the Equatorial Channel at 35°W. The other part recirculates and some of it flows through the Romanche Fracture Zone into the eastern Atlantic.[11]
In the Guiana Basin, west of 40°W, the sloping topography and the strong, eastward flowing deep western boundary current might prevent the Antarctic bottom water from flowing west: thus it has to turn north at the eastern slope of the Ceará Rise. At 44°W, north of the Ceará Rise, Antarctic bottom water flows west in the interior of the basin. A large fraction of the Antarctic bottom water enters the eastern Atlantic through the Vema Fracture Zone.[11]

Indian Ocean
In the Indian Ocean the Crozet-Kerguelen Gap allows Antarctic bottom water to move toward the equator. This northward movement amounts to 2.5 Sv. It takes the Antarctic Bottom Water 23 years to reach the Crozet-Kerguelen Gap.[12] South of Africa, Antarctic bottom water flow northwards through the Agulhas Basin and then east through the Agulhas Passage and over the southern margins of the Agulhas Plateau from where it is transported to into the Mozambique Basin.[13]
Climate change
Climate change and the subsequent melting of the Southern ice sheet have slowed the formation of AABW, and this slowdown is likely to continue. A complete shutdown of AABW formation is possible as soon as 2050.[14] This shutdown would have dramatic effects on ocean circulation and global weather patterns.
Potential for AABW Disruption
Due to the cold temperatures and high density of AABW, changes coming from increased surface water temperatures and increased ice melt can impact how the water flows.[15] For surface water to become deep water, it must be very cold and saline. Much of the deep-water formation comes from brine rejection where the water deposited is extremely saline and cold, making it extremely dense. The increased ice melt that occurred starting in the early 2000s has created a period of fresher water between 2011-2015 within the bottom water.[16] This has been distinctly prevalent in Antarctic bottom waters near West Antarctica primarily in the Weddell Sea area.[16]
While the freshening of the AABW has corrected itself over the past few years with a decrease in ice melt, the potential for more ice melt in the future still poses a threat.[16] With the potential increase in ice melt at extreme enough levels, it can have a serious impact on the ability for deep sea water to be formed. While this would create a slowdown referenced above, it may also create additional warming. Increased stratification coming from the fresher and warmer waters will reduce bottom and deep-water circulation and increase warm water flows around Antarctica.[15] The sustained warmer surface waters would only increase the level of ice melt, stratification, and the slowdown of the AABW circulation and formation. Additionally, without the presence of those colder waters producing brine rejection which deposits to the AABW, there may eventually be no formation of bottom water around Antarctica anymore.[15] This would impact more than Antarctica as AABW plays a major role in bottom water formation and deep-sea circulation which deposits oxygen to the deep sea and is a major carbon sink. Without these connections, the deep sea will become drastically changed with the potential for collapse in entire deep-sea communities.[15]
Some studies indicate that WSBW formation in the Weddell Sea is dominantly driven by wind-driven sea ice changes, however, and that increased sea ice formation overcompensates for the melting of ice sheets, rendering the effects of melting Antarctic glaciers on WSBW minimal.[17]
References
- ↑ Schmidt, Christina; Morrison, Adele K.; England, Matthew H. (17 June 2023). "Wind– and Sea-Ice–Driven Interannual Variability of Antarctic Bottom Water Formation". Journal of Geophysical Research: Oceans. 128 (6). doi:10.1029/2023JC019774. S2CID 259468175.
- ↑ "AMS Glossary of Meteorology, Antarctic Bottom Water". American Meteorological Society. Retrieved 29 June 2023.
- ↑ Gunn, Kathryn L.; Rintoul, Stephen R.; England, Matthew H.; Bowen, Melissa M. (June 2023). "Recent reduced abyssal overturning and ventilation in the Australian Antarctic Basin". Nature Climate Change. 13 (6): 537–544. doi:10.1038/s41558-023-01667-8.
- ↑ Talley, Lynne (1999). "Some aspects of ocean heat transport by the shallow, intermediate and deep overturning circulations". Mechanisms of Global Climate Change at Millennial Time Scales. Geophysical Monograph Series. Vol. 112. pp. 1–22. Bibcode:1999GMS...112....1T. doi:10.1029/GM112p0001. ISBN 0-87590-095-X.
- ↑ Massom, R.; Michael, K.; Harris, P.T.; Potter, M.J. (1998). "The distribution and formative processes of latent heat polynyas in East Antarctica". Annals of Glaciology. 27: 420–426. Bibcode:1998AnGla..27..420M. doi:10.3189/1998aog27-1-420-426.
- ↑ Broecker, W. S.; Peacock, S. L.; Walker, S.; Weiss, R.; Fahrbach, E.; Schroeder, M.; Mikolajewicz, U.; Heinze, C.; Key, R.; Peng, T.-H.; Rubin, S. (1998). "How much deep water is formed in the Southern Ocean?". Journal of Geophysical Research: Oceans. 103 (C8): 15833–15843. Bibcode:1998JGR...10315833B. doi:10.1029/98JC00248.
- ↑ Kusahara, Kazuya; Hasumi, Hiroyasu; Williams, Guy D. (2011). "Impact of the Mertz Glacier Tongue calving on dense water formation and export". Nature Communications. 2 (1): 159. Bibcode:2011NatCo...2..159K. doi:10.1038/ncomms1156. PMID 21245840.
- ↑ Harris, P.T. (2000). "Ripple cross-laminated sediments on the East Antarctic shelf: evidence for episodic bottom water production during the Holocene?". Marine Geology. 170 (3–4): 317–330. Bibcode:2000MGeol.170..317H. doi:10.1016/s0025-3227(00)00096-7.
- ↑ Harris, P.T.; Brancolini, G.; Armand, L.; Busetti, M.; Beaman, R.J.; Giorgetti, G.; Prestie, M.; Trincardi, F. (2001). "Continental shelf drift deposit indicates non-steady state Antarctic bottom water production in the Holocene". Marine Geology. 179 (1–2): 1–8. Bibcode:2001MGeol.179....1H. doi:10.1016/s0025-3227(01)00183-9.
- ↑ "AMS Glossary, Vema Channel". American Meteorological Society. Retrieved 2012-02-20.
- 1 2 Rhein, Monika; Stramma, Lothar; Krahmann, Gerd (1998). "The spreading of Antarctic bottom water in the tropical Atlantic" (PDF). Deep-Sea Research Part I. 45 (4–5): 507–527. Bibcode:1998DSRI...45..507R. CiteSeerX 10.1.1.571.6529. doi:10.1016/S0967-0637(97)00030-7. Retrieved 2012-02-14.
- ↑ Haine, T. W. N.; Watson, A. J.; Liddicoat, M. I.; Dickson, R. R. (1998). "The flow of Antarctic bottom water to the southwest Indian Ocean estimated using CFCs". Journal of Geophysical Research. 103 (C12): 27637–27653. Bibcode:1998JGR...10327637H. doi:10.1029/98JC02476.
- ↑ Uenzelmann-Neben, G.; Huhn, K. (2009). "Sedimentary deposits on the southern South African continental margin: Slumping versus non-deposition or erosion by oceanic currents?" (PDF). Marine Geology. 266 (1–4): 65–79. Bibcode:2009MGeol.266...65U. doi:10.1016/j.margeo.2009.07.011. Retrieved 1 April 2015.
- ↑ Hansen, James; Sato, Makiko; Hearty, Paul; Ruedy, Reto; Kelley, Maxwell; Masson-Delmotte, Valerie; Russell, Gary; Tselioudis, George; Cao, Junji (2016-03-22). "Ice melt, sea level rise and superstorms: evidence from paleoclimate data, climate modeling, and modern observations that 2 °C global warming could be dangerous". Atmospheric Chemistry and Physics. 16 (6): 3761–3812. arXiv:1602.01393. Bibcode:2016ACP....16.3761H. doi:10.5194/acp-16-3761-2016. ISSN 1680-7324. S2CID 9410444.
- 1 2 3 4 Silvano, A., Rintoul, S. R., Peña-Molino, B., Hobbs, W. R., van Wijk, E., Aoki, S., ... & Williams, G. D. (2018). Freshening by glacial meltwater enhances the melting of ice shelves and reduces the formation of Antarctic Bottom Water. Science advances, 4(4), eaap9467.
- 1 2 3 Aoki, S., Yamazaki, K., Hirano, D., Katsumata, K., Shimada, K., Kitade, Y., ... & Murase, H. (2020). Reversal of freshening trend of Antarctic Bottom Water in the Australian-Antarctic Basin during 2010s. Scientific reports, 10(1), 1-7.
- ↑ Zhou, Shenjie; Meijers, Andrew J. S.; Meredith, Michael P.; Abrahamsen, E. Povl; Holland, Paul R.; Silvano, Alessandro; Sallée, Jean-Baptiste; Østerhus, Svein (12 June 2023). "Slowdown of Antarctic Bottom Water export driven by climatic wind and sea-ice changes". Nature Climate Change. 13 (7): 701–709. doi:10.1038/s41558-023-01695-4. ISSN 1758-6798.
- Glossary of Physical Oceanography
- Steele, John H., Steve A. Thorpe and Karl K. Turekian, editors, Ocean Currents: A derivative of the Encyclopedia of Ocean Sciences, Academic Press, 1st ed., 2010 ISBN 978-0-08-096486-7
- Seabrooke, James M.; Hufford, Gary L.; Elder, Robert B. (1971). "Formation of Antarctic Bottom Water in the Weddell Sea". Journal of Geophysical Research. 76 (9): 2164–2178. Bibcode:1971JGR....76.2164S. doi:10.1029/jc076i009p02164.
- Fahrbach, E.; Rohardt, G.; Scheele, N.; Schroder, M.; Strass, V.; Wisotzki, A. (1995). "Formation and discharge of deep and bottom water in the northwestern Weddell Sea". Journal of Marine Research. 53 (4): 515–538. doi:10.1357/0022240953213089.