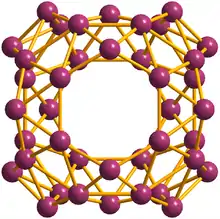
40° borospherene
Borospherene (B40) is an electron-deficient cluster molecule containing 40 boron atoms. It bears similarities to other homoatomic cluster strucrures such as buckminsterfullerene (C60), stannaspherene, and plumbaspherene, but with a different symmetry.[1][2][3] The first experimental evidence for borospherene was reported in July 2014, and is described in the journal Nature Chemistry.[4] The molecule includes unusual hexagonal and heptagonal faces. Despite many calculation-based investigations into its structure and properties, a viable route for the synthesis and isolation of borospherene has yet to be established, and as a consequence it is still relatively poorly understood.
History

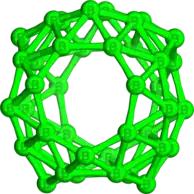
In 2014, the first experimental evidence of a homoelemental fullerene-like B40 cluster was reported by Zhai et al., after decades of theoretical investigations into boron cage structures following the discovery of buckminsterfullerene.[5] Anionic B40- clusters were transiently produced by laser vaporisation of a 10B-enriched boron disc target, and studied with photoelectron spectroscopy. Their experimental spectrum corresponded well to a combination of simulated spectra of a sheet-like, quasi-planar global minimum of the B40- anion (Cs symmetry) and its nearly degenerate fullerene-like structural isomer (D2d symmetry).
Many theoretical papers have been published on the structure, properties, and potential applications of borospherene. Neutral borospherene has a large HOMO-LUMO gap of 3.13 eV (which destabilises its anion, making the ground state of B40- the quasi-planar isomer). However, it has been calculated to be prone to exothermic dimerisation, with a low activation barrier of 63 meV, followed by trimerisation with a lower energy barrier, and runaway aggregation.[6] As a result, borospherene has yet to be isolated and is poorly experimentally-characterised, unlike buckminsterfullerene.
Structure
Borospherene has a unique C2 axis of symmetry, and belongs to the symmetry group is D2d (antiprismatic symmetry, like a baseball) - in contrast to buckminsterfullerene, which has icosahedral symmetry. It features eight close-packed B6 triangles, two staggered hexagonal holes at its top and bottom, as well as four heptagonal holes along its sides.[5][7] Unusually, the heptagons induce positive Gaussian curvature (as opposed to negative Gaussian curvature in carbon nanotubes), which may play a role in strain reduction contributing to the stability of the cluster.[8]
16 boron atoms of borospherene are four-coordinate, and 24 are five-coordinate. It has four sets of eight equivalent boron atoms, and two sets of four equivalent atoms.
.png.webp)
.png.webp)
Neutral borospherene has a diameter of 6.2 Å. It comprises eleven unique bond lengths ranging from 1.60 Å to 1.85 Å, corresponding to a B-B bond order of slightly below 2 to a fractional B-B bond order respectively. This encapsulates well the large degree of both sigma- and pi-delocalisation of electrons across the electron-deficient cluster as opposed to buckminsterfullerene, which has more localised bonds and features only two bond lengths corresponding to a C-C single bond and a C-C double bond respectively. The HOMO of borospherene is quadruply degenerate, computed to be a pi-bond delocalised over 5 boron atoms.
Lai-Sheng Wang, professor of chemistry at Brown University, modeled possible B40 and B40- anion structures. The simulated spectra of two energetically lowest-lying isomers of the anion - a sheet-like structure and a closed cage - were found to fit experimental data well. Photoelectron spectroscopy revealed that the substance formed in the laboratory was this cage. Both neutral borospherene and the cage-like isomer of its anion have the same D2d symmetry, the additional electron in the anion being housed within the B40- cage structure.[1] The structure of the cage is not perfectly uniform – "Several atoms stick out a bit from the others, making the surface of borospherene somewhat less smooth than a buckyball" according to Wang.[1]
Potential applications
The cavity within the cage-like structure of borospherene, as well as borospherene's coordinatively unsaturated hexagonal and heptagonal faces, allows for the possibility of its endohedral or exohedral doping.[9][10] With metal dopants, significant charge transfer is calculated to occur from the metals to the B40 cage - resulting in a positive charge forming on the metal, ostensibly making it capable of polarising small molecules. Such complexes formed are theorised to have applications in catalysis, and the detection or storage of small molecules such as H2.[11]
Small molecule sensing
Exploiting the thermal stability of B40 (calculated to be stable up to 1000 K), Liu et al. investigated, with Van der Waals-corrected density functional theory calculations, the feasibility of using alkali metal-decorated B40 for the reversible storage and optical detection of hydrogen.[12] Optimisation of (AM)6B40 structures (AM = Li, Na, K) revealed the metal atoms to be distributed above the centres of each hexagon and heptagon of B40, with a large binding energy in each case suggesting these complexes should be stable. H2 adsorption to these complexes induced a red-shift in their simulated TDDFT optical spectra in the case of Li6B40, and a blue-shift in the cases of Na6B40 and K6B40.
Li et al. computationally investigated undecorated borospherene as a potential sensor for sulfur-containing gases, and found that it behaved as an electronic sensor for sulfur dioxide and carbon disulfide (their adsorption to the boron cluster significantly stabilises its LUMO, increasing its population of conducting electrons), and additionally as a Φ-type sensor for the former (due to significant change to its work function Φ upon the adsorption of SO2), but behaved as neither for the gases carbonyl sulfide and hydrogen sulfide.[13]
Small molecule storage
Modelling an exohedral Ca6B40, Esrafili et al. simulated carbon dioxide adsorption to the complex and found the upper bound of adsorption to be four CO2 molecules per Ca, with an average binding energy of -0.54 eV each - falling within the optimal range of binding energies for a CO2 adsorbent (0.40 - 0.80 eV), allowing facile desorption at elevated temperatures.[14]
Undecorated B40 was calculated to be a poor candidate for reversible hydrogen storage, being capable of the irreversible sequestration of only one hydrogen molecule per B40 within its cage. Li6B40, however, is calculated to be capable of adsorbing up to 18 H2 molecules (3 H2 molecules at each Li site) - corresponding to a gravimetric density of 7.1 wt% - with a moderate average binding energy of 0.11 eV/H2, within the optimal range for reversible hydrogen storage. Subsequent H2 molecules are physisorbed to the cluster instead of chemisorbed, and have a much weaker binding energy.[15]
See also
References
- 1 2 3 Stacey, Kevin. "Researchers discover boron "buckyball"". news.brown.edu. Brown University. Retrieved 14 July 2014.
- ↑ Cui, Li-Feng; Xin Huang; Lei-Ming Wang; Dmitry Yu. Zubarev; Alexander I. Boldyrev; Jun Li; Lai-Sheng Wang (2006-07-01). "Sn122-: Stannaspherene". Journal of the American Chemical Society. 128 (26): 8390–8391. doi:10.1021/ja062052f. ISSN 0002-7863. PMID 16802791.
- ↑ Cui, Li-Feng; Xin Huang; Lei-Ming Wang; Jun Li; Lai-Sheng Wang (2006-08-01). "Pb122-: Plumbaspherene". The Journal of Physical Chemistry A. 110 (34): 10169–10172. Bibcode:2006JPCA..11010169C. doi:10.1021/jp063617x. ISSN 1089-5639. PMID 16928103.
- ↑ Zhai, Hua-Jin; Ya-Fan Zhao; Wei-Li Li; Qiang Chen; Hui Bai; Han-Shi Hu; Zachary A. Piazza; Wen-Juan Tian; Hai-Gang Lu; Yan-Bo Wu; Yue-Wen Mu; Guang-Feng Wei; Zhi-Pan Liu; Jun Li; Si-Dian Li; Lai-Sheng Wang (2014-07-13). "Observation of an all-boron fullerene". Nature Chemistry. advance online publication (8): 727–731. Bibcode:2014NatCh...6..727Z. doi:10.1038/nchem.1999. ISSN 1755-4349. PMID 25054944.
- 1 2 Zhai, Hua-Jin; Ya-Fan Zhao; Wei-Li Li; Qiang Chen; Hui Bai; Han-Shi Hu; Zachary A. Piazza; Wen-Juan Tian; Hai-Gang Lu; Yan-Bo Wu; Yue-Wen Mu; Guang-Feng Wei; Zhi-Pan Liu; Jun Li; Si-Dian Li; Lai-Sheng Wang (2014-07-13). "Observation of an all-boron fullerene". Nature Chemistry. advance online publication (8): 727–731. Bibcode:2014NatCh...6..727Z. doi:10.1038/nchem.1999. ISSN 1755-4349. PMID 25054944.
- ↑ Yang, Yang; Zhang, Zhuhua; Penev, Evgeni S.; Yakobson, Boris I. (2017-02-02). "B40 cluster stability, reactivity, and its planar structural precursor". Nanoscale. 9 (5): 1805–1810. doi:10.1039/C6NR09385J. ISSN 2040-3372.
- ↑ Quick, Darren (July 14, 2014). "Borospherene bounces into buckyball family". Gizmag.com. Retrieved 2014-07-14.
- ↑ Yang, Yang; Zhang, Zhuhua; Penev, Evgeni S.; Yakobson, Boris I. (2017-02-02). "B40 cluster stability, reactivity, and its planar structural precursor". Nanoscale. 9 (5): 1805–1810. doi:10.1039/C6NR09385J. ISSN 2040-3372.
- ↑ Bai, Hui; Chen, Qiang; Zhai, Hua‐Jin; Li, Si‐Dian (2015-01-12). "Endohedral and Exohedral Metalloborospherenes: M@B 40 (M=Ca, Sr) and M&B 40 (M=Be, Mg)". Angewandte Chemie International Edition. 54 (3): 941–945. doi:10.1002/anie.201408738. ISSN 1433-7851.
- ↑ Yu, Tianrong; Gao, Yang; Xu, Dexuan; Wang, Zhigang (2018-01-01). "Actinide endohedral boron clusters: A closed-shell electronic structure of U@B40". Nano Research. 11 (1): 354–359. doi:10.1007/s12274-017-1637-9. ISSN 1998-0000.
- ↑ Kaur, Harleen; Kaur, Jupinder; Kumar, Ravinder (2022-01-01). "A review on all boron fullerene (B40): A promising material for sensing and device applications". Materials Today: Proceedings. SCPINM-2021. 48: 1095–1102. doi:10.1016/j.matpr.2021.07.465. ISSN 2214-7853.
- ↑ Liu, Chun-Sheng; Ye, Xiao-Juan; Wang, Xiangfu; Yan, Xiaohong (2016-06-13). "Metalized B40 fullerene as a novel material for storage and optical detection of hydrogen: a first-principles study". RSC Advances. 6 (62): 56907–56912. doi:10.1039/C6RA08378A. ISSN 2046-2069.
- ↑ Li, Changyu; Tang, Wenxiu; Vahabi, Vahid (2020-06-01). "Identification of sulfur gases by an B40 fullerene: A computational study". Physica E Low-Dimensional Systems and Nanostructures. 120: 114038. doi:10.1016/j.physe.2020.114038. ISSN 1386-9477.
- ↑ Esrafili, Mehdi D.; Mousavian, Parisasadat (2021-10-16). "Ca coated B40 fullerene: A promising material for CO2 storage and separation". Chemical Physics Letters. 781: 138991. doi:10.1016/j.cplett.2021.138991. ISSN 0009-2614.
- ↑ Bai, Hui; Bai, Bing; Zhang, Lin; Huang, Wei; Mu, Yue-Wen; Zhai, Hua-Jin; Li, Si-Dian (2016-10-18). "Lithium-Decorated Borospherene B40: A Promising Hydrogen Storage Medium". Scientific Reports. 6 (1): 35518. doi:10.1038/srep35518. ISSN 2045-2322. PMC 5067665.
External links

Media related to Borospherene at Wikimedia Commons