In 2015, the UN defined a new sustainability-focused development plan based on 17 sustainable development goals. It recognized the need of "green chemistry" and "green solvent" for a more sustainable chemistry in the future. At the same time, more and more companies want to go green, to ensure that their activities and products are part of a sustainable development process. It is in this context that so-called green, ecological, biodegradable, and sustainable solvents have emerged.[1], which were developed as a more environmentally friendly solvents, or biosolvents, derived from the processing of agricultural crops, an alternative to petrochemical solvents.
Background
A solvent is a substance that is liquid at the temperature at which it is used. It has the ability to dissolve, dilute or extract other substances without changing them chemically and without changing itself. The traditional organic solvents (acetone, NMP, toluene, etc.), although very effective, raise today many problems as they are associated with adverse effects, both on the health and safety of workers (burns, cancer, eczema, brain disorder (encephalopathy), fetotoxicity (via the placenta), and inflammations of several peripheral nerves at the same time, as well as on the environment and health of the general population (smog precursors, water, air and ozone layer pollution). Solvents represent a major part of the chemical use in various domains (paints, coating, synthesis, ...) and in consequence define a lot of the environmental performance of the chemical industry.
Classification
Whereas regular solvents are easily classified in two categories being polar and nonpolar, it is much harder to do so for green solvents as their chemical structure and source can differ a lot. In fact, green solvents are established from trials and errors in search for substitutes of existing hazardous solvents.
Here is a non-exhaustive list of substances that research have found advantages in favor of being qualified as green solvents, based on their production method, or the raw materials from which they are produced:
Water
Water is not an organic solvent because it contains no carbon atoms, and it is the first green solvent that comes to mind when thinking of solvent-solute mixtures. Water is a polar protic solvent thanks to its chemical structure, non-toxic and renewable. For instance in living matter, ions and proteins are all dissolved in water. It is the cheapest and most abundant solvent for a large range of reactions and processes in industrial chemistry. There are cases where traditional organic solvents can be replaced by aqueous preparations.[2] Water-based coatings have largely replaced petroleum-based paints for the construction industry. However, traditional solvent-based anti corrosion paints remain the most used today.
Supercritical fluid
Some substances that occur in the gas phase at ambient temperature and pressure can act as solvents if heated to temperatures and pressures above their critical value. This is an area where the gas and liquid states exist in a single phase with properties intermediate between liquid and gas, including the mobility of gas and the dissolving power of liquid: a supercritical fluid.
- Supercritical water (SCW) is obtained at a temperature of 374.2 °C and a pressure of 22.05 MPa.[3] It behaves as a dense gas with a dissolving power equivalent to that of organic solvents of low polarity. However, the solubility of inorganic salts in SCW is radically reduced. SCW is used as a reaction medium, especially in oxidation processes for the destruction of toxic substances such as those found in industrial aqueous effluents. The use of supercritical water has two main technical challenges, namely corrosion and salt deposition.
- Supercritical CO2 Carbon dioxide (CO2) is the most commonly used supercritical fluid because of the relatively easy to reach conditions. Temperatures above 31 °C and pressures above 7.38 MPa are sufficient.[4] It then behaves as a good apolar solvent.
Solvents derived from carbohydrates
- Ethanol is the second most used solvent after water. Thus, it is found used in toiletries, cosmetics, some cleaners and coatings. Studies show that simple alcohols such as methanol and ethanol are to be preferred environmentally, unlike formaldehyde or dioxane. The results of this case study indicate that methanol-water or ethanol-water mixtures are environmentally friendly compared to pure alcohol or propanol-water mixtures.

- Ethyl lactate, made from lactic acid obtained from corn starch, is notably used as a mixture with other solvents in some paint strippers and cleaners.[5] Ethyl lactate has replaced solvents such as toluene, acetone, and xylene, resulting in a much safer workplace.
- Bioethanol is made industrially by fermentation of sugars and starch, one of the oldest known chemical processes, but also from cellulose.
- Biobutanol (butyl alcohol, various isomers) is also produced by fermentation of sugars. Isobutanol and tert-butanol are used as solvents in paints.
- Tetrahydrofurfuryl alcohol (THFA) is obtained from hemicellulose.
Solvents derived from lipids

Lipids (triglycerides) themselves can be used as solvents. But they are mostly hydrolyzed to fatty acids and glycerol (glycerin). Fatty acids can be esterified with an alcohol to give fatty acid esters, e.g., FAMEs (fatty acid methyl ester) if the esterification is performed with methanol. Usually derived from natural gas or petroleum, the methanol used to produce FAMEs can also be obtained by other routes, including thermochemical gasification of biomass and household hazardous waste. Glycerol from lipid hydrolysis can be used as a solvent in synthetic chemistry as some of its derivatives.[7]
Deep eutectic solvents
By combining certain substances in given proportions, it is possible to obtain a mixture whose melting point is lower than that of the constituents: this is called an eutectic mixture. Many solid substances mixed in this way become liquids that can be used as solvents, especially when the melting-point depression is very large, hence the term deep eutectic solvent (DES). One of the most commonly used substances to obtain DES is the quaternary ammonium salt called choline chloride (trimethyl hydroxyethylammonium chloride). Smith, Abbott, and Ryder report that a mixture of urea (melting point: 133 °C) and choline chloride (melting point: 302 °C) in a 2:1 molar ratio has a melting point of 12 °C.[1]
Natural deep eutectic solvents (NADES) are also a research area, the latter being easy to produce from only two low-cost and well-known ecotoxicity components, a hydrogen-bond acceptor and a hydrogen-bond donor).[8]
Solvents obtained by extraction
-Limonen2.svg.png.webp)
Solvents in a diverse class of natural substances called terpenes are obtained by extraction from certain parts of plants. All terpenes are structurally presented as multiples of isoprene with the gross formula (C5H8)n. D-limonene, a monoterpene, is one of the best known solvents in this class, as is turpentine. D-limonene is extracted from citrus peels while turpentine is obtained from pine trees (sap, stump) and as a by-product of the Kraft paper-making process (Sell, 2006).[9] Turpentine is a mixture of terpenes whose composition varies according to its origin and production method. In Canada and the United States, a range of mass concentrations of 40 to 65% α-pinene and 20 to 35% β-pinene, but also 2 to 20% d-limonene are found.[10] α-pinene can replace n-hexane for the extraction of vegetable oil and as a substitute solvent for extracting molecules such as carotenoids used especially as food additives.[11] Turpentine, formerly used as a solvent in organic coatings, is now largely replaced by petroleum hydrocarbons.[10] Nowadays, it is mainly used as a source of its constituents, including α-pinene and β-pinene.[12]
Ionic liquids

Ionic liquids are molten organic salts that are generally fluid at room temperature. The most popular cations, variously substituted, include imidazolium, pyridinium, ammonium and phosphonium. Anions include halides, tetrafluoroborate, hexafluorophosphate, and nitrate. Bubalo et al. (2015)[13] argue that ionic liquids are non-flammable, chemically, electrochemically and thermally stable, with negligible volatility. Indeed, they are named green solvents, as their low volatility allows them to limit VOC emissions compared to conventional solvents. Optimistically, ionic liquids from renewable and biodegradable materials have recently emerged. But their ecotoxicity and poor degradability had been recognized in the past because the resources typically used for their production are non-renewable, as is the case for imidazole and halogenated alkanes, derived from petroleum. Plus, their availability is up for debate because their production cost is high.[8]
Switchable solvents
Bubbling CO2 into water or an organic solvent results in changes to certain properties of the liquid such as its polarity, ionic strength, and hydrophilicity. As an example, this allows an organic solvent to form a homogeneous mixture with otherwise immiscible water. Furthermore, the process is reversible. Jessop et al. (2012) developed this technology which has the potential to be used in synthetic chemistry, extraction and separation of various substances. The degree of how green this technology is, is measured by the energy and material savings it provides; thus, one of the advantages of switchable solvents is the reuse of solvent and water[14]
Solvents from waste materials
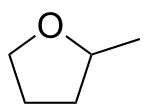
1st generation biorefineries exploit food-based substances such as starch and vegetable oils.[15] For example, corn grain is used to make ethanol. Second-generation biorefineries use residues or wastes generated by various industries as feedstock for the manufacture of their solvents. 2-Methyltetrahydrofuran, derived from lignocellulosic waste, would have the potential to replace tetrahydrofuran, toluene, DCM, and diethyl ether in some applications. Levulinic acid esters from the same source would have the potential to replace DCM in paint cleaners and strippers.
Used cooking oils can be used to produce FAMEs (Byrne, F. et al., 2017).[16] Glycerol, obtained as a byproduct of the synthesis of these, can in turn be used to produce various solvents such as 2,2-dimethyl-1,3-dioxolane-4-methanol, usable as a solvent in the formulation of inks and cleaners[17]
Fusel oil, a mixture of amyl alcohol isomers, is a byproduct of ethanol production from sugars as seen previously. Green solvents derived from fusel oil such as isoamyl acetate or isoamyl methyl carbonate could be obtained . When used to manufacture nail polishes, VOC emissions report a minimum reduction of 68% compared to the emissions by using traditional solvents.
Petrochemical solvents with certain green characteristics
Notably due to the high price of new sustainable solvents, Clark et al. (2017)[18] list twenty-five solvents that are currently considered acceptable to replace hazardous solvents even if they are petrochemical derived.

These include propylene carbonate and dibasic esters (DBEs). Propylene carbonate and DBEs have been the subject of monographs on solvent substitution.[19][20] Propylene carbonate and two DBEs are considered green in the manufacturer GlaxoSmithKline's (GSK) Solvent Sustainability Guide used in the pharmaceutical industry.[21] Propylene carbonate can also be produced from renewable resources. On the other hand, the DBEs that have appeared on the market in recent years are more and more obtained by valorizing by-products of the synthesis of polyamides, derived from petroleum. Other petrochemical solvents are variously referred to as green solvents, such as certain halogenated hydrocarbons like parachlorobenzotrifluoride (PCBTF). This one has been used since the early 1990s in paints to replace smog-forming solvents.
Siloxanes are compounds containing silicon, oxygen, carbon and hydrogen atoms. They are known in industry, especially in the form of polymers (silicones, R-SiO-R'), for their thermal stability and elastic and non-stick properties. The early 1990s saw the emergence of low molecular weight siloxanes (methylsiloxanes), which can be used as solvents in precision cleaning, replacing stratospheric ozone-depleting solvents.
A final category of petrochemical solvents that qualify as green involves polymeric solvents. The International Union of Pure and Applied Chemistry (IUPAC), defines the term polymer solvent as follows: a polymer that acts as a solvent for low-molecular weight compounds.[22] In industrial chemistry, polyethylene glycols (PEGs, H(OCH2CH2)nOH) are one of the most widely used polymeric solvent families.[23] PEGs, with molecular weights below 600 Da, are viscous liquids at room temperature while heavier PEGs are waxy solids.
Soluble in water and readily biodegradable, liquid PEGs have the advantage of negligible volatility (< 0.01 mmHg or < 1.3 Pa at 20 °C)...[24] PEGs are synthesized from ethylene glycol and ethylene oxide, both of which are petrochemicals derived molecules. Ethylene glycol from renewable sources (cellulose) is commercially available.[25]
Physical properties
The physical properties of solvents are important in identifying the solvent used according to the reaction conditions. In particular, their dissolution properties make it possible to assess the use of a particular solvent for a chemical reaction, such as an extraction or a washing. Evaporation is also important to consider as it tells about the potential emission of volatile organic compound (VOC).
The following table shows some properties of green solvents in each category.
Classification | Solvent | Molar mass
(g·mol−1) |
Solubility in water (at 25 °C) | Melting point (°C) | Boiling point (°C) | Density (g/mL) | Dielectric constant | Appearance | Vapor pressure | Viscosity |
---|---|---|---|---|---|---|---|---|---|---|
Water | Water[26][27][28] | 18 | 0 | 100 | 1.000 at 3.98 °C | 78.304 at 25 °C | colorless | 23.8 mmHg at 25 °C | 0.8949 cP·s at 25 °C | |
Solvents derived from carbohydrates | Ethyl Lactate[29][30][31] | 118.13 | Fully Miscible | –26 | 154 | 1.03 | 15.7 | colorless | 0.22 kPa
at 20 °C 17 kPa at 100 °C |
0.428 cP·s at 25 °C |
Ethanol[32][33] | 46.07 | 106 mg/mL | –114.14 | 78.24 | 0.7893 at 20 °C | 24.5 | colorless | 10 kPa at 29.2 °C | 1.074 mPa·s at 25 °C | |
Solvents from waste materials | 2-Methyltetrahydrofuran[34] | 86.13 | 150 g/L | –136 | 80 | 0.85 at 20 °C | colorless | 13.6 kPa at 20 °C
34,5kPa at 50 °C |
4 mPa·s at 25 °C | |
Levulinic acid[35][36][37][38][39][40] | 116.11 | 6.746·105 mg/L | 33 | 245.5 | 1.134 at 25 °C | solid: large crystal
liquid: yellow to brown |
2.984 kPa at 156.85 °C | 14.26 mPa·s at 40 °C | ||
Solvents obtained by extraction | Limonene[41][42][43][44] | 136.23 | 14 mg/L | −74.35 | 177~178 | 0.8402 at 20.85 °C | 2.3746 | colorless | 1.55 mmHg
at 25 °C |
0.897 mPa·s
at 25.15 °C |
Petrochemical solvents | Propylene Carbonate[45][46] | 102.09 | 1.75·105 mg/L | –48.8 | 241.6 | 1.204 at 25 °C | colorless | 0.13 mmHg at 20 °C |
For the other categories, some precisions can be developed.
Deep eutectic solvents (DES)[47] have huge hydrogen bonds, leading them to have lower melting points. Some of them have lower melting points than 50 °C and they are investigated because they can be cheap, safe and useful in industries. Most of the DES have a higher density than water but its range is difficult to grasp as it depends on the components from which they are synthesized and their molecular arrangement and vacancies. For example, Octylammonium bromide/Decanoic acid (molar ratio of [1:2]) has a lower density compared to water of 0.8889 g.cm−3, up to 1.4851 g.cm−3 for Choline chloride/Trifluoroacetamine [1:2]. Their miscibility is also composition-dependent.
Fatty acid methyl esters[48][49][50]. They are investigated and compared to fossil diesel. At 20 °C or 40 °C, those solvents have a lower density than water at 4 °C (temperature in which the water is the densest):
: from 0.9079 (acetate) to 0.8488 (arachidate) and : from 0.9338 (acetate) to 0.8663 (pentadecanoate).
Their kinematic viscosity depends if they are saturated or unsaturated or even the temperature. At 40 °C, for saturated FAMEs, it goes from 0.340 (acetate) to 6.39 (nonadecanoate), and for unsaturated FAMEs, it goes from 5.61 for the stearate to 7.21 for the erucate.
Their dielectric constant decreases as their alkyl chain gets longer. For example, acetate has a tiny alkyl chain and has a dielectric constant of ε40= 6.852 and ε40= 2.982 for the nonadecanoate.
Switchable solvents[51][52]. Their switchable properties can be caused by the strength of their conjugate acid's pKa and octanol-water partition coefficient ratio Kow. They must have a pKa above 9.5 to be protonated by carbonated water and also a log(Kow) between 1.2 and 2.5 to be switchable because they will not be too hydrophilic or hydrophobic. It depends on the volumetric ratio of the compound compared to water, so volumetric ratios influence their switchable properties. For example, N,N,N′-Tributylpentanamidine is switchable, and for a volumetric ratio of compound to water of 2:1, it has a log(Kow)= 5.99, which is higher than 2.5. It can be explained by the fact that the volume of the compound is twice higher than the water volume.
Ionic liquids[53]. Scientists have identified that ionic liquids with lower melting point were asymmetric cations and high melting point are associated with symmetric cations. If they have branched alkyl chains they will have a higher melting point.
About their density, they are more dense than water, going from 1.05 to 1.64 g·cm−3 at 20 °C and it decrease to values from 1.01 to 1.57 at 90 °C.
Applications
Conforming to their various chemical nature, green solvents come to use for applications in a lot of domains. Not only are they preferred for their low impact on the environment, but they have been found to have other pros such as more efficient physicochemical properties or reaction yield than when using traditional solvents. However, the results obtained are for the most part observations from experiments on particular green solvents, so they cannot yet be generalized.
Organic synthesis
Green solvents efficiency has mainly been proven in extractions and separations in comparison to traditional solvents.[54]
- Supercritical CO2 is largely used in the food industry as an extraction solvent. Among other processes like flavoring agents, fragrances, essential oils, or lipid extraction from plants, sc-CO2 is a green substitute to dichloromethane in coffee decaffeination, avoiding the use of a hazardous solvent and additional synthesis steps. Sc-CO2 can also apply to polymerization reactions, specifically in PTFE (polytetrafluoroethylene) formation to manipulate monomers safely and avoid explosive reactions of peroxide with dioxygen. Although the original process involves water, a green solvent itself, sc-CO2 allows less waste materials, thus reducing the E factor,[55] indicator of how "green" a reaction is.
- Observations report that the higher the deep eutectic solvent's hydrophobicity, the higher the extraction efficiency of neonicotinoids from aqueous solutions, although the exact trend has not been established yet.[56] In fact, the creation of a biphasic system is easier to achieve. In 2015 for the first time, several hydrophobic DES composed of highly hydrophobic hydrogen bond donors have been reported, one of them being decanoic acid with a quaternary ammonium salt, and a fatty acid as a hydrogen bond acceptor.[47]
- The pharmaceutical industry intends to substitute their solvents for greener options, all the more because solvent use in active substance synthesis is important, and downgrades solvents with a high boiling point. That is explained because the solvent must generally be evaporated at the end of a chemical reaction, hence the insistence on low-boiling solvents in order to minimize the energy required for its removal by distillation.[57]
Industrial chemistry
- Ethyle lactate has an excellent cleaning power, resulting in various use to clean metal surfaces, to remove greases, oils, adhesives and solid fuels. That explains their role in aqueous preparations used for industrial degreasing, but also in coatings, adhesives and inks.
- Fatty acid methyl esters (FAMEs), through studies, have been used as a reactive diluent in coatings for continuous metal strip coating[58] (e.g., the interior coating of food cans), reducing the amount of volatile solvent in this type of coating, and so doing, lowering their toxicity at work and for the environment.
- Tetrahydrofurfuryl alcohol (THFA) mixtures with other green solvents are studied for their cleaning properties. As an example, the mixture of THFA with FAME and ethyl lactate has been patented as a paint stripper.[59]
- Ionic liquids particularly have applications in electrodeposition.[60] Their relevance as green solvents is further enhanced by the emergence of production methods based on renewable and biodegradable resources[61]
On another note, solvent manufacturers provide industrial companies with softwares that use a database to propose green alternative solvent mixtures to those originally used in industrial processes, which conclude to similar efficiency and reaction yield. However, environmental and safety requirements are not always considered in those suggestions.[62]
Safety
The use of green solvents is increasingly privileged because of their lower environmental impact. But as any chemical product, these solvents present dangers for human health as well as for the environment. However, for a number of green solvents, their impact is still unclear, or at least, not categorized yet.
Here are listed some information from safety data sheets of common green solvents[63][64]
Solvents | Pictograms | Hazards |
---|---|---|
Ethyl Lactate | ![]() ![]() ![]() |
Flammable liquid and vapors
Causes severe eye damage. May irritate the respiratory tract |
Ethanol | ![]() ![]() |
Highly flammable liquid and vapors
Causes severe eye irritation |
2-Methyltetrahydrofuran | ![]() ![]() ![]() |
Highly flammable liquid and vapors
Harmful if swallowed. Causes skin irritation and severe eye damage |
Levulinic acid | ![]() |
Harmful if swallowed. Causes skin irritation
Causes severe eye irritation |
Limonene | ![]() ![]() ![]() ![]() |
Flammable liquid and vapors
Causes skin irritation. May cause skin allergy Very toxic to aquatic organisms, causes long-term adverse effects |
Propylene Carbonate | ![]() |
Harmful or irritating
Causes severe eye irritation |
Water. Under normal conditions, water toxicity is rare because there is a precise regulation of the body's water content.
Ethyl lactate. On acute exposure, it causes irritation of the ocular and respiratory mucosa and is a mild skin irritant. No data are available on chronic exposure, carcinogenicity, genotoxicity and reproductive toxicity in humans.
Ethanol. High concentrations of ethanol cause central nervous system depressant effects associated with severe eye and upper airway irritation that is rapidly intolerable. Splashing in the eye results in reversible conjunctivitis. Repeated exposure may result in eye and airway irritation associated with mild neurological impairment. The IARC classified in 2007 "ethanol in alcoholic beverages" in group 1 of carcinogens for humans.
2-Methyltetrahydrofuran. During an acute exposure, it cause skin irritation and severe eye damage. However, it is not classified as a respiratory or skin sensitizer, nor is it a germ cell mutagen. This solvent is also not classified as a carcinogen or reproductive toxicant.
Levulinic acid. This solvent is relatively non-toxic. Although it causes severe eye damage, and allergic skin reactions, it is not classified as a germ cell mutagen, carcinogen, or reproductive toxin.
Limonene. Ingestion of limonene may cause digestive irritation and reversible proteinuria without renal damage. The inhalation of vapours or aerosols can cause irritation of the upper airways and eyes. Depending on the concentration, digestive disorders and CNS damage are possible. It also causes irritation of the skin and eye mucosa. Dipentene does not appear to have significant chronic toxicity, except for irritating and sensitizing effects. No carcinogenic effects have been reported in humans.
Propylene carbonate. It is considered one of the least dangerous solvents, because apart from causing severe eye irritation, it is not a respiratory or skin sensitizer. In addition, it is not classified as a germ cell mutagen, carcinogen, or reproductive toxin.
Legislation
Environmental issues have recently become more important. Green chemistry issues are even more so. We do not have a sufficient amount of experimental data on the impact of solvent greens on health or on the environment. That is why there is not yet much legislation on them.
Solvents derived from carbohydrates
For ethanol, the American Conference of Governmental Industrial Hygienists, shortened ACGIH, advises a short-term exposure limit of 1000 ppm to avoid irritating the respiratory tract.[65]
The French National Agency for Food, Environmental, and Occupational Health Safety (ANSES) has recommended a short-term occupational exposure limit value of 100 mg/m3 for butan-1-ol, a solvent used in paints, cleaners, and degreasers, in order to prevent irritation of the mucous membranes of the eyes and upper airways. Since 1998, the ACGIH has suggested an 8 hour exposure limit value (ELV) of 20 ppm of butan-1-ol to prevent irritation of the upper respiratory tract and eyes.
Male rats exposed to THFA develop reproductive toxicity. Moreover, it has an impact on fetal and embryonic development in rats. The American Industrial Hygiene Association suggested an ELV of 2 ppm for THFA to prevent testicular degeneration in 1993 based on the No-observed-effect level of two subchronic investigations in rats and dogs.
Deep Eutectic Solvents
DES components, according to Wazeer, Hayyan, and Hadj-Kali,[66] are typically non-toxic and biodegradable. According to Hayyan et al.[67], the DES they investigated were more harmful to the small crustacean artemia than each of their individual components, which could be attributed to synergy. The abbreviation NADES refers to DES that contain only materials sourced from renewable resources. Compared to other DES, these would typically be less hazardous.
Solvents from extraction
Turpentine irritates the skin, eyes, and upper respiratory system. It also increases skin sensitivity. Furthermore, it is regarded in the Regulation of occupational health and safety as such.
Petrochemical solvents
Polyethylene glycols (PEG) irritate the skin and eyes on a weak basis. For PEG aerosols, the American Industrial Hygiene Association has recommended an occupational exposure limit value of 10 mg/m3.
References
- 1 2 Denis Bégin; Caroline Couture; Michel Gérin; Maximilien Debia (2020). Solvants verts: fondements, santé, sécurité, environnement et substitution (in French). Montréal, QC, CA: Université de Montréal. Département de santé environnementale et santé au travail, IRSST, Bibliothèque numérique canadienne. ISBN 9782897971113. OCLC 1162187204.
- ↑ Lavoué, J.; Bégin, D.; Géerin, M. (2003). "Technical, Occupational Health and Environmental Aspects of Metal Degreasing with Aqueous Cleaners". The Annals of Occupational Hygiene. 47 (6): 441–459. doi:10.1093/annhyg/meg057. ISSN 1475-3162. PMID 12890654.
- ↑ Martín, Ángel; José Cocero, María (2016). "Supercritical Fluids". Kirk-Othmer Encyclopedia of Chemical Technology. Hoboken, NJ, USA: John Wiley & Sons. pp. 1–28. doi:10.1002/0471238961.1921160504092415.a01.pub3. ISBN 9780471238966.
- ↑ Lumia, Guy (2002). "Utilisation du CO2 supercritique comme solvant de substitution". Environnement. doi:10.51257/a-v1-in5. S2CID 171743208.
- ↑ "Institut de Recherche Robert-Sauvé en Santé et en Sécurité du Travail (IRSST)", The Grants Register 2021, London: Palgrave Macmillan UK, pp. 452–453, 2020, doi:10.1057/978-1-349-95988-4_465, ISBN 978-1-349-95987-7
- ↑ Saxena, Neha; Pal, Nilanjan; Ojha, Keka; Dey, Swapan; Mandal, Ajay (2018-07-02). "Synthesis, characterization, physical and thermodynamic properties of a novel anionic surfactant derived from Sapindus laurifolius". RSC Advances. 8 (43): 24485–24499. Bibcode:2018RSCAd...824485S. doi:10.1039/C8RA03888K. ISSN 2046-2069. PMC 9082051. PMID 35539155.
- ↑ Gu, Yanlong; Jérôme, François (2013). "Bio-based solvents: an emerging generation of fluids for the design of eco-efficient processes in catalysis and organic chemistry". Chemical Society Reviews. 42 (24): 9550–9570. doi:10.1039/c3cs60241a. ISSN 0306-0012. PMID 24056753.
- 1 2 Winterton, Neil (2021). "The green solvent: a critical perspective". Clean Technologies and Environmental Policy. 23 (9): 2499–2522. doi:10.1007/s10098-021-02188-8. ISSN 1618-9558. PMC 8482956. PMID 34608382. S2CID 238233639.
- ↑ Sell, Charles S. (2006). "Terpenoids". Kirk-Othmer Encyclopedia of Chemical Technology. Hoboken, NJ, USA: John Wiley & Sons. doi:10.1002/0471238961.2005181602120504.a01.pub2. ISBN 0471238961.
- 1 2 Gscheidmeier, Manfred; Fleig, Helmut (2000). "Turpentines". Ullmann's Encyclopedia of Industrial Chemistry. Weinheim, Germany: Wiley-VCH Verlag GmbH & Co. KGaA. doi:10.1002/14356007.a27_267. ISBN 978-3-527-30673-2.
- ↑ Boutekedjiret, Chahrazed; Vian, Maryline Abert; Chemat, Farid (2014), "Terpenes as Green Solvents for Natural Products Extraction", Alternative Solvents for Natural Products Extraction, Green Chemistry and Sustainable Technology, Berlin, Heidelberg: Springer Berlin Heidelberg, pp. 205–219, doi:10.1007/978-3-662-43628-8_9, ISBN 978-3-662-43627-1
- ↑ "Turpentine (8006-64-2)", Chemical Effects in Biological Systems (CEBS), Research Triangle Park, NC (USA): National Toxicology Program (NTP), 2017, doi:10.22427/NTP-DATA-DTXSID6027717
- ↑ Cvjetko Bubalo, Marina; Vidović, Senka; Radojčić Redovniković, Ivana; Jokić, Stela (2015). "Green solvents for green technologies". Journal of Chemical Technology & Biotechnology. 90 (9): 1631–1639. doi:10.1002/jctb.4668. ISSN 0268-2575. S2CID 97363599.
- ↑ Jessop, Philip G.; Mercer, Sean M.; Heldebrant, David J. (2012). "CO2-triggered switchable solvents, surfactants, and other materials". Energy & Environmental Science. 5 (6): 7240–7253. doi:10.1039/C2EE02912J. ISSN 1754-5706.
- ↑ Bayart, Marie; Foruzanmehr, M. Reza; Vuillaume, Pascal Y.; Ovlaque, Pierre; Robert, Mathieu; Elkoun, Saïd (2021). "Poly(lactic acid)/flax composites: effect of surface modification and thermal treatment on interfacial adhesion, crystallization, microstructure, and mechanical properties". Composite Interfaces. 29 (1): 17–36. doi:10.1080/09276440.2021.1884470. ISSN 0927-6440. S2CID 233830208.
- ↑ Jin, Saimeng; Byrne, Fergal; McElroy, Con Robert; Sherwood, James; Clark, James H.; Hunt, Andrew J. (2017). "Challenges in the development of bio-based solvents: a case study on methyl(2,2-dimethyl-1,3-dioxolan-4-yl)methyl carbonate as an alternative aprotic solvent" (PDF). Faraday Discussions. 202: 157–173. Bibcode:2017FaDi..202..157J. doi:10.1039/c7fd00049a. ISSN 1359-6640. PMID 28661518.
- ↑ Byrne, Fergal P.; Jin, Saimeng; Paggiola, Giulia; Petchey, Tabitha H. M.; Clark, James H.; Farmer, Thomas J.; Hunt, Andrew J.; Robert McElroy, C.; Sherwood, James (2016). "Tools and techniques for solvent selection: green solvent selection guides". Sustainable Chemical Processes. 4 (1). doi:10.1186/s40508-016-0051-z. ISSN 2043-7129. S2CID 56045622.
- ↑ Sustainable Solvents. Green Chemistry Series. 2017. doi:10.1039/9781782624035. ISBN 978-1-78262-335-9. ISSN 1757-7047.
- ↑ "Institut de Recherche Robert-Sauvé en Santé et en Sécurité du Travail (IRSST)", The Grants Register 2022, London: Palgrave Macmillan UK, pp. 494–495, 2021, doi:10.1057/978-1-349-96042-2_6517, ISBN 978-1-349-96041-5
- ↑ Bisson Desrochers, Alexandra; Rouleau, Isabelle; Angehrn, Andréanne; Vasiliadis, Helen-Maria; Saumier, Daniel; Brunet, Alain (2021). "Trauma on duty: cognitive functioning in police officers with and without posttraumatic stress disorder (PTSD)". European Journal of Psychotraumatology. 12 (1). doi:10.1080/20008198.2021.1959117. ISSN 2000-8066. PMC 8555514. PMID 34721819.
- ↑ Alder, Catherine M.; Hayler, John D.; Henderson, Richard K.; Redman, Anikó M.; Shukla, Lena; Shuster, Leanna E.; Sneddon, Helen F. (2016). "Updating and further expanding GSK's solvent sustainability guide". Green Chemistry. 18 (13): 3879–3890. doi:10.1039/c6gc00611f. ISSN 1463-9262.
- ↑ Horie, K.; Barón, Máximo; Fox, R. B.; He, J.; Hess, M.; Kahovec, J.; Kitayama, T.; Kubisa, P.; Maréchal, E.; Mormann, W.; Stepto, R. F. T.; Tabak, D.; Vohlídal, J.; Wilks, E. S.; Work, W. J. (2004). "Definitions of terms relating to reactions of polymers and to functional polymeric materials (IUPAC Recommendations 2003)". Pure and Applied Chemistry. 76 (4): 889–906. doi:10.1351/pac200476040889. ISSN 1365-3075. S2CID 98351038.
- ↑ Clarke, Coby J.; Tu, Wei-Chien; Levers, Oliver; Bröhl, Andreas; Hallett, Jason P. (2018). "Green and Sustainable Solvents in Chemical Processes". Chemical Reviews. 118 (2): 747–800. doi:10.1021/acs.chemrev.7b00571. hdl:10044/1/59694. ISSN 0009-2665. PMID 29300087.
- ↑ Technical study for the chemical cleaning of Dresden-1. Volume I, Section 1 and 2 (Report). 1977. doi:10.2172/5335747. OSTI 5335747.
- ↑ Zhu, Yunqing; Romain, Charles; Williams, Charlotte K. (2016). "Sustainable polymers from renewable resources". Nature. 540 (7633): 354–362. Bibcode:2016Natur.540..354Z. doi:10.1038/nature21001. hdl:10044/1/37073. ISSN 0028-0836. PMID 27974763. S2CID 205253152.
- ↑ "Water". PubChem. National Center for Biotechnology Information.
- ↑ Malmberg, C.G.; Maryott, A.A. (1956). "Dielectric constant of water from 0° to 100 °C". Journal of Research of the National Bureau of Standards. 56 (1): 1. doi:10.6028/jres.056.001. ISSN 0091-0635.
- ↑ "The Drive to Make Things Happen". Virtual Chemistry Laboratory. 2005.
- ↑ Kua, Yin Leng; Gan, Suyin; Morris, Andrew; Ng, Hoon Kiat (2016). "Ethyl lactate as a potential green solvent to extract hydrophilic (polar) and lipophilic (non-polar) phytonutrients simultaneously from fruit and vegetable by-products". Sustainable Chemistry and Pharmacy. 4: 21–31. doi:10.1016/j.scp.2016.07.003. ISSN 2352-5541.
- ↑ "Lactate d'éthyle - Fiche toxicologique n° 240". Institut National de Recherche et Sécurité (INRS) (in French). 2001.
- ↑ Chen, Jui-Tang; Chu, Hsiao-Pei (2007). "Densities and Viscosities for Binary Mixtures of Ethyl Lactate with Methacrylic Acid, Benzyl Methacrylate, and 2-Hydroxyethyl Methacrylate at (298.15, 308.15, and 318.15) K". Journal of Chemical & Engineering Data. 52 (2): 650–654. doi:10.1021/je600568w. ISSN 0021-9568.
- ↑ "Annotation Record for Ethanol". PubChem Hazardous Substances Data Bank (HSDB). National Center for Biotechnology Information.
- ↑ Sierra-Amor, Rosa (2001). "CRC Handbook of Laboratory Safety, 5th ed. A. Keith Furr, ed. Boca Raton, FL: CRC Press LCC, 2000, 774 pp., $149.99". Clinical Chemistry. 47 (11): 2075. doi:10.1093/clinchem/47.11.2075a. ISBN 0-8493-2523-4. ISSN 0009-9147.
- ↑ Institut für Arbeitsschutz der Deutschen Gesetzlichen Unfallversicherung (IFA) (2016). "2-Methyltetrahydrofuran anhydrous". GESTIS-Stoffdatenbank.
- ↑ Kumar, Anuj; Shende, Diwakar Z.; Wasewar, Kailas L. (2020). "Extractive separation of levulinic acid using natural and chemical solvents". Chemical Data Collections. 28: 100417. doi:10.1016/j.cdc.2020.100417. S2CID 219461679.
- ↑ "Levulinic acid". PubChem. National Center for Biotechnology Information.
- ↑ Ariba, Houda; Wang, Yanjun; Devouge-Boyer, Christine; Stateva, Roumiana P.; Leveneur, Sébastien (2020). "Physicochemical Properties for the Reaction Systems: Levulinic Acid, Its Esters, and γ-Valerolactone" (PDF). Journal of Chemical & Engineering Data. 65 (6): 3008–3020. doi:10.1021/acs.jced.9b00965. ISSN 0021-9568. S2CID 219417194.
- ↑ Wohlfarth, Christian (2016), Lechner, M. D. (ed.), "Surface tension of levulinic acid", Surface Tension of Pure Liquids and Binary Liquid Mixtures, Berlin, Heidelberg: Springer Berlin Heidelberg, p. 86, doi:10.1007/978-3-662-48336-7_83, ISBN 978-3-662-48335-0
- ↑ Agrawal, Kushagra; Chakraborty, Pritam; Kishore, Nanda (2020). "Thermochemical Mapping of Levulinic Acid Conversion to Pentane in Supercritical Water within the Framework of Density Functional Theory". Energy & Fuels. 34 (9): 11061–11072. doi:10.1021/acs.energyfuels.0c01906. ISSN 0887-0624. S2CID 225513425.
- ↑ Stull, Daniel R. (1947). "Vapor Pressure of Pure Substances. Organic and Inorganic Compounds". Industrial & Engineering Chemistry. 39 (4): 517–540. doi:10.1021/ie50448a022. ISSN 0019-7866.
- ↑ Clará, René A.; Marigliano, Ana C. Gómez; Sólimo, Horacio N. (2009). "Density, Viscosity, and Refractive Index in the Range (283.15 to 353.15) K and Vapor Pressure of α-Pinene, d -Limonene, (±)-Linalool, and Citral Over the Pressure Range 1.0 kPa Atmospheric Pressure". Journal of Chemical & Engineering Data. 54 (3): 1087–1090. doi:10.1021/je8007414. ISSN 0021-9568.
- ↑ "Limonene". PubChem. National Center for Biotechnology Information.
- ↑ "Limonene". American Chemical Society. 2021.
- ↑ Thomas, Gerald A.; Hawkins, J. Erskine (1954). "Physical and Thermodynamic Properties of Terpenes. IV. The Dielectric Constant, Refractive Index and Density of Some Terpenes 1". Journal of the American Chemical Society. 76 (19): 4856–4858. doi:10.1021/ja01648a026. ISSN 0002-7863.
- ↑ Wang, Xiangyu; Pan, Hongwei; Jia, Shiling; Lu, Zifeng; Han, Lijing; Zhang, Huiliang (2023). "Mechanical properties, thermal behavior, miscibility and light stability of the poly(butylene adipate-co-terephthalate)/poly(propylene carbonate)/polylactide mulch films". Polymer Bulletin. 80 (3): 2485–2501. doi:10.1007/s00289-022-04173-7. ISSN 0170-0839. S2CID 247408345.
- ↑ "Propylene Carbonate". PubChem. National Center for Biotechnology Information.
- 1 2 Omar, Karzan A.; Sadeghi, Rahmat (2022). "Physicochemical properties of deep eutectic solvents: A review". Journal of Molecular Liquids. 360: 119524. doi:10.1016/j.molliq.2022.119524. S2CID 249384715.
- ↑ Gouw, T. H.; Vlugter, J. C. (1964). "Physical properties of fatty acid methyl esters. I. density and molar volume". Journal of the American Oil Chemists' Society. 41 (2): 142–145. doi:10.1007/BF02673494. S2CID 85252625.
- ↑ Gouw, T. H.; Vlugter, J. C.; Roelands, C. J. A. (1966). "Physical properties of fatty acid methyl esters. VI. Viscosity". Journal of the American Oil Chemists' Society. 43 (7): 433–434. doi:10.1007/BF02682408. S2CID 85301006.
- ↑ Gouw, T. H.; Vlugter, J. C. (1964). "Physical properties of fatty acid methyl esters. V. Dielectric constant". Journal of the American Oil Chemists' Society. 41 (10): 675–678. doi:10.1007/BF02661406. S2CID 86752228.
- ↑ Jessop, Philip G.; Phan, Lam; Carrier, Andrew; Robinson, Shona; Dürr, Christoph J.; Harjani, Jitendra R. (2010). "A solvent having switchable hydrophilicity". Green Chemistry. 12 (5): 809. doi:10.1039/b926885e. ISSN 1463-9262.
- ↑ Vanderveen, Jesse R.; Durelle, Jeremy; Jessop, Philip G. (2014). "Design and evaluation of switchable-hydrophilicity solvents". Green Chem. 16 (3): 1187–1197. doi:10.1039/C3GC42164C. ISSN 1463-9262.
- ↑ Braunstein, J.; Mamantov, Gleb; Smith, G. P. (1973). Advances in Molten Salt Chemistry : Volume 2. Boston, MA: Springer US. ISBN 978-1-4757-0662-8. OCLC 853263478.
- ↑ Clarke, Coby J.; Tu, Wei-Chien; Levers, Oliver; Bröhl, Andreas; Hallett, Jason P. (2018). "Green and Sustainable Solvents in Chemical Processes". Chemical Reviews. 118 (2): 747–800. doi:10.1021/acs.chemrev.7b00571. hdl:10044/1/59694. ISSN 0009-2665. PMID 29300087.
- ↑ Sheldon, Roger A. (2000). "Atom utilisation, E factors and the catalytic solution". Comptes Rendus de l'Académie des Sciences - Series IIC - Chemistry. 3 (7): 541–551. doi:10.1016/S1387-1609(00)01174-9. ISSN 1387-1609.
- ↑ Osch, Dannie J. G. P. van; Zubeir, Lawien F.; Bruinhorst, Adriaan van den; Rocha, Marisa A. A.; Kroon, Maaike C. (2015). "Hydrophobic deep eutectic solvents as water-immiscible extractants". Green Chemistry. 17 (9): 4518–4521. doi:10.1039/C5GC01451D. ISSN 1463-9270. S2CID 94513163.
- ↑ Byrne, Fergal P.; Jin, Saimeng; Paggiola, Giulia; Petchey, Tabitha H. M.; Clark, James H.; Farmer, Thomas J.; Hunt, Andrew J.; Robert McElroy, C.; Sherwood, James (2016). "Tools and techniques for solvent selection: green solvent selection guides". Sustainable Chemical Processes. 4 (1): 7. doi:10.1186/s40508-016-0051-z. ISSN 2043-7129. S2CID 56045622.
- ↑ Johansson, Katarina; Johansson, Mats K. G. (2007). "Fatty Acid Methyl Esters as Reactive Diluents in Coil-Coatings". Polymer Preprints. 48 (2): 857–858.
- ↑ Bergemann, E. P., Opre, J. E. et Henneberry, M. (2000). "Environmentally friendly solvent". Patent n°6,096,699. Washington, DC: United States Patent and Trademark Office
- ↑ Frank Endres; Andrew Abbott; Douglas MacFarlane, eds. (2017). Electrodeposition from ionic liquids (2nd ed.). Weinheim, Germany. ISBN 978-3-527-68270-6. OCLC 973412068.
{{cite book}}
: CS1 maint: location missing publisher (link) - ↑ Hulsbosch, Joris; De Vos, Dirk E.; Binnemans, Koen; Ameloot, Rob (2016). "Biobased Ionic Liquids: Solvents for a Green Processing Industry?". ACS Sustainable Chemistry & Engineering. 4 (6): 2917–2931. doi:10.1021/acssuschemeng.6b00553. ISSN 2168-0485.
- ↑ Wiersma, R. (2014). "Formula for success in developing advanced solvent blends". Polymer Paint Colour Journal - PPCJ, 204 (4592), 54-55.
- ↑ "Information en matière de sécurité donnée (CE) N°1907, 2006 (REACH)" (PDF). Carl Roth. 2021.
- ↑ "Fiches toxicologiques". Publications et outils (in French). Institut National de Recherche et de Sécurité (INRS).
- ↑ 2018 TLVs and BEIs: based on the documentation of the threshold limit values for chemical substances and physical agents & biological exposure indices. Cincinnati, OH: American Conference of Governmental Industrial Hygienists. 2018. ISBN 978-1-60726-097-4. OCLC 1029463040.
- ↑ Wazeer, Irfan; Hayyan, Maan; Hadj-Kali, Mohamed K (2017). "Deep eutectic solvents: designer fluids for chemical processes". Journal of Chemical Technology & Biotechnology. 93 (4): 945–958. doi:10.1002/jctb.5491. ISSN 0268-2575.
- ↑ Hayyan, Maan; Hashim, Mohd Ali; Hayyan, Adeeb; Al-Saadi, Mohammed A.; AlNashef, Inas M.; Mirghani, Mohamed E.S.; Saheed, Olorunnisola Kola (2013). "Are deep eutectic solvents benign or toxic?". Chemosphere. 90 (7): 2193–2195. Bibcode:2013Chmsp..90.2193H. doi:10.1016/j.chemosphere.2012.11.004. ISSN 0045-6535. PMID 23200570.