In physical chemistry and engineering, passivation is coating a material so that it becomes "passive", that is, less readily affected or corroded by the environment. Passivation involves creation of an outer layer of shield material that is applied as a microcoating, created by chemical reaction with the base material, or allowed to build by spontaneous oxidation in the air. As a technique, passivation is the use of a light coat of a protective material, such as metal oxide, to create a shield against corrosion.[1] Passivation of silicon is used during fabrication of microelectronic devices.[2] Undesired passivation of electrodes, called "fouling", increases the circuit resistance so it interferes with some electrochemical applications such as electrocoagulation for wastewater treatment, amperometric chemical sensing, and electrochemical synthesis.[3]
When exposed to air, many metals naturally form a hard, relatively inert surface layer, usually an oxide (termed the "native oxide layer") or a nitride, that serves as a passivation layer. In the case of silver, the dark tarnish is a passivation layer of silver sulfide formed from reaction with environmental hydrogen sulfide. (In contrast, metals such as iron oxidize readily to form a rough porous coating of rust that adheres loosely and sloughs off readily, allowing further oxidation.) The passivation layer of oxide markedly slows further oxidation and corrosion in room-temperature air for aluminium, beryllium, chromium, zinc, titanium, and silicon (a metalloid). The inert surface layer formed by reaction with air has a thickness of about 1.5 nm for silicon, 1–10 nm for beryllium, and 1 nm initially for titanium, growing to 25 nm after several years. Similarly, for aluminium, it grows to about 5 nm after several years.[4][5][6]
In the context of the semiconductor device fabrication, such as silicon MOSFET transistors and solar cells, surface passivation refers not only to reducing the chemical reactivity of the surface but also to eliminating the dangling bonds and other defects that form electronic surface states, which impair performance of the devices. Surface passivation of silicon usually consists of high-temperature thermal oxidation.
Mechanisms
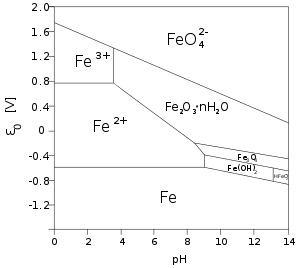
There has been much interest in determining the mechanisms that govern the increase of thickness of the oxide layer over time. Some of the important factors are the volume of oxide relative to the volume of the parent metal, the mechanism of oxygen diffusion through the metal oxide to the parent metal, and the relative chemical potential of the oxide. Boundaries between micro grains, if the oxide layer is crystalline, form an important pathway for oxygen to reach the unoxidized metal below. For this reason, vitreous oxide coatings – which lack grain boundaries – can retard oxidation.[8] The conditions necessary, but not sufficient, for passivation are recorded in Pourbaix diagrams. Some corrosion inhibitors help the formation of a passivation layer on the surface of the metals to which they are applied. Some compounds, dissolved in solutions (chromates, molybdates) form non-reactive and low solubility films on metal surfaces.
History
Discovery and etymology
The fact that iron doesn't react with concentrated nitric acid was discovered by Mikhail Lomonosov in 1738 and rediscovered by James Keir in 1790, who also noted that such pre-immersed Fe doesn't reduce silver from nitrate anymore.[9] In the 1830s, Michael Faraday and Christian Friedrich Schönbein studied that issue systematically and demonstrated that when a piece of iron is placed in dilute nitric acid, it will dissolve and produce hydrogen, but if the iron is placed in concentrated nitric acid and then returned to the dilute nitric acid, little or no reaction will take place. In 1836, Schönbein named the first state the active condition and the second the passive condition while Faraday proposed the modern explanation of the oxide film described above (Schönbein disagreed with it), which was experimentally proven by Ulick Richardson Evans only in 1927.[9]
Specific materials
Aluminium
Aluminium naturally forms a thin surface layer of aluminium oxide on contact with oxygen in the atmosphere through a process called oxidation, which creates a physical barrier to corrosion or further oxidation in many environments. Some aluminium alloys, however, do not form the oxide layer well, and thus are not protected against corrosion. There are methods to enhance the formation of the oxide layer for certain alloys. For example, prior to storing hydrogen peroxide in an aluminium container, the container can be passivated by rinsing it with a dilute solution of nitric acid and peroxide alternating with deionized water. The nitric acid and peroxide mixture oxidizes and dissolves any impurities on the inner surface of the container, and the deionized water rinses away the acid and oxidized impurities.[10]
Generally, there are two main ways to passivate aluminium alloys (not counting plating, painting, and other barrier coatings): chromate conversion coating and anodizing. Alclading, which metallurgically bonds thin layers of pure aluminium or alloy to different base aluminium alloy, is not strictly passivation of the base alloy. However, the aluminium layer clad on is designed to spontaneously develop the oxide layer and thus protect the base alloy.
Chromate conversion coating converts the surface aluminium to an aluminium chromate coating in the range of 0.00001–0.00004 inches (250–1,000 nm) in thickness. Aluminium chromate conversion coatings are amorphous in structure with a gel-like composition hydrated with water.[11] Chromate conversion is a common way of passivating not only aluminium, but also zinc, cadmium, copper, silver, magnesium, and tin alloys.
Anodizing is an electrolytic process that forms a thicker oxide layer. The anodic coating consists of hydrated aluminium oxide and is considered resistant to corrosion and abrasion.[12] This finish is more robust than the other processes and also provides electrical insulation, which the other two processes may not.
Carbon
In carbon quantum dot (CQD) technology, CQDs are small carbon nanoparticles (less than 10 nm in size) with some form of surface passivation.[13][14][15]
Ferrous materials

Ferrous materials, including steel, may be somewhat protected by promoting oxidation ("rust") and then converting the oxidation to a metalophosphate by using phosphoric acid and add further protection by surface coating. As the uncoated surface is water-soluble, a preferred method is to form manganese or zinc compounds by a process commonly known as parkerizing or phosphate conversion. Older, less effective but chemically similar electrochemical conversion coatings included black oxidizing, historically known as bluing or browning. Ordinary steel forms a passivating layer in alkali environments, as reinforcing bar does in concrete.
Stainless steel
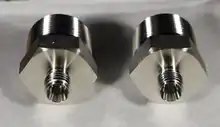
Stainless steels are corrosion-resistant, but they are not completely impervious to rusting. One common mode of corrosion in corrosion-resistant steels is when small spots on the surface begin to rust because grain boundaries or embedded bits of foreign matter (such as grinding swarf) allow water molecules to oxidize some of the iron in those spots despite the alloying chromium. This is called rouging. Some grades of stainless steel are especially resistant to rouging; parts made from them may therefore forgo any passivation step, depending on engineering decisions.[16]
Common among all of the different specifications and types are the following steps: Prior to passivation, the object must be cleaned of any contaminants and generally must undergo a validating test to prove that the surface is 'clean.' The object is then placed in an acidic passivating bath that meets the temperature and chemical requirements of the method and type specified between customer and vendor. While nitric acid is commonly used as a passivating acid for stainless steel, citric acid is gaining in popularity as it is far less dangerous to handle, less toxic, and biodegradable, making disposal less of a challenge. Passivating temperatures can range from ambient to 60 °C (140 °F), while minimum passivation times are usually 20 to 30 minutes. After passivation, the parts are neutralized using a bath of aqueous sodium hydroxide, then rinsed with clean water and dried. The passive surface is validated using humidity, elevated temperature, a rusting agent (salt spray), or some combination of the three.[17] The passivation process removes exogenous iron,[18] creates/restores a passive oxide layer that prevents further oxidation (rust), and cleans the parts of dirt, scale, or other welding-generated compounds (e.g. oxides).[18][19]
Passivation processes are generally controlled by industry standards, the most prevalent among them today being ASTM A 967 and AMS 2700. These industry standards generally list several passivation processes that can be used, with the choice of specific method left to the customer and vendor. The "method" is either a nitric acid-based passivating bath, or a citric acid-based bath, these acids remove surface iron and rust, while sparing the chromium. The various 'types' listed under each method refer to differences in acid bath temperature and concentration. Sodium dichromate is often required as an additive to oxidise the chromium in certain 'types' of nitric-based acid baths, however this chemical is highly toxic. With citric acid, simply rinsing and drying the part and allowing the air to oxidise it, or in some cases the application of other chemicals, is used to perform the passivation of the surface.
It is not uncommon for some aerospace manufacturers to have additional guidelines and regulations when passivating their products that exceed the national standard. Often, these requirements will be cascaded down using Nadcap or some other accreditation system. Various testing methods are available to determine the passivation (or passive state) of stainless steel. The most common methods for validating the passivity of a part is some combination of high humidity and heat for a period of time, intended to induce rusting. Electro-chemical testers can also be utilized to commercially verify passivation.
Titanium

The surface of titanium and of titanium-rich alloys oxidizes immediately upon exposure to air to form a thin passivation layer of titanium oxide, mostly titanium dioxide.[20] This layer makes it resistant to further corrosion, aside from gradual growth of the oxide layer, thickening to ~25 nm after several years in air. This protective layer makes it suitable for use even in corrosive environments such as sea water. Titanium can be anodized to produce a thicker passivation layer. As with many other metals, this layer causes thin-film interference which makes the metal surface appear colored, with the thickness of the passivation layer directly effecting the color produced.
Nickel
Nickel can be used for handling elemental fluorine, owing to the formation of a passivation layer of nickel fluoride. This fact is useful in water treatment and sewage treatment applications.
Silicon
In the area of microelectronics and photovoltaic solar cells, surface passivation is usually implemented by thermal oxidation at about 1000 °C to form a coating of silicon dioxide. Surface passivation is critical to solar cell efficiency.[21] The effect of passivation on the efficiency of solar cells ranges from 3–7%. The surface resistivity is high, > 100 Ωcm.[22]
Perovskite
The easiest and most widely studied method to improve perovskite solar cells is passivation. These defects usually lead to deep energy level defects in solar cells due to the presence of hanging bonds on the surface of perovskite films.[23][24] Usually, small molecules or polymers are doped to interact with the hanging bonds and thus reduce the defect states. This process is similar to Tetris, i.e., we always want the layer to be full. A small molecule with the function of passivation is some kind of square that can be inserted where there is an empty space and then a complete layer is obtained. These molecules will generally have lone electron pairs or pi-electrons, so they can bind to the defective states on the surface of the cell film and thus achieve passivation of the material. Therefore, molecules such as carbonyl,[25] nitrogen-containing molecules,[26] and sulfur-containing molecules[27] are considered, and recently it has been shown that Pi electrons can also play a role.[28]
In addition, passivation not only improves the photoelectric conversion efficiency of perovskite cells, but also contributes to the improvement of device stability. For example, adding a passivation layer of a few nanometers thickness can effectively achieve passivation with the effect of stopping water vapor intrusion.[29]
See also
References
- ↑ "Passivation vs Electropolishing – What are the differences?". electro-glo.com. 10 June 2019. Retrieved 6 February 2022.
- ↑ IUPAC Goldbook
- ↑ Yang, Xiaoyun; Kirsch, Jeffrey; Fergus, Jeffrey; Simonian, Aleksandr (2013). "Modeling analysis of electrode fouling during electrolysis of phenolic compounds". Electrochimica Acta. 94: 259–268. doi:10.1016/j.electacta.2013.01.019. ISSN 0013-4686.
- ↑ "Semiconductor Glossary". semi1source.com. Retrieved 6 February 2022.
- ↑ Bockris & Reddy 1977, p. 1325
- ↑ Fehlner, Francis P (1986). Low-Temperature Oxidation: The Role of Vitreous Oxides, A Wiley-Interscience Publication. New York: John Wiley & Sons. ISBN 0471-87448-5.
- ↑ University of Bath Archived 3 March 2009 at the Wayback Machine & Western Oregon University
- ↑ Fehlner, Francis P, ref.3.
- 1 2 Lu, Xinying (10 February 2023). Passivation and Corrosion of Black Rebar with Mill Scale. Springer Nature. ISBN 978-981-19-8102-9.
- ↑ Aluminum Passivation
- ↑ Chemical Conversion Coating on Aluminum
- ↑ Aluminum Anodizing Process Archived 20 March 2019 at the Wayback Machine
- ↑ Wang, Youfu; Hu, Aiguo (2014). "Carbon quantum dots: Synthesis, properties and applications". Journal of Materials Chemistry C. 2 (34): 6921–39. doi:10.1039/C4TC00988F.
- ↑ Fernando, K. A. Shiral; Sahu, Sushant; Liu, Yamin; Lewis, William K.; Guliants, Elena A.; Jafariyan, Amirhossein; Wang, Ping; Bunker, Christopher E.; Sun, Ya-Ping (2015). "Carbon Quantum Dots and Applications in Photocatalytic Energy Conversion". ACS Applied Materials & Interfaces. 7 (16): 8363–76. doi:10.1021/acsami.5b00448. PMID 25845394.
- ↑ Gao, Xiaohu; Cui, Yuanyuan; Levenson, Richard M; Chung, Leland W K; Nie, Shuming (2004). "In vivo cancer targeting and imaging with semiconductor quantum dots". Nature Biotechnology. 22 (8): 969–76. doi:10.1038/nbt994. PMID 15258594. S2CID 41561027.
- ↑ "Stainless Steel Passivation". Arrow Cryogenics. Archived from the original on 4 March 2014. Retrieved 28 February 2014.
- ↑ "Carpenter Technical Articles – HOW TO PASSIVATE STAINLESS STEEL PARTS". Archived from the original on 22 October 2013. Retrieved 8 May 2013.
- 1 2 "Stainless Steel Passivation Services – A967 & A380 | Delstar Metal Finishing, Inc".
- ↑ "Pickling and Passivating Stainless Steel" (PDF). Euro Inox. Archived from the original (PDF) on 12 September 2012. Retrieved 1 January 2013.
- ↑ Chen, George Zheng; Fray, Derek J.; Farthing, Tom W. (2001). "Cathodic deoxygenation of the alpha case on titanium and alloys in molten calcium chloride". Metallurgical and Materials Transactions B. 32 (6): 1041–1052. doi:10.1007/s11663-001-0093-8. ISSN 1073-5615. S2CID 95616531.
- ↑ Black, Lachlan E. (2016). New Perspectives on Surface Passivation: Understanding the Si-Al2O3 Interface (PDF). Springer. ISBN 9783319325217.
- ↑ Aberle, Armin G. (2000). "Surface passivation of crystalline silicon solar cells: A review". Progress in Photovoltaics: Research and Applications. 8 (5): 473–487. doi:10.1002/1099-159X(200009/10)8:5<473::AID-PIP337>3.0.CO;2-D.
- ↑ Stranks, Samuel (2020). "Performance-limiting nanoscale trap clusters at grain junctions in halide perovskites". Nature. 580 (7803): 360–366. Bibcode:2020Natur.580..360D. doi:10.1038/s41586-020-2184-1. PMID 32296189. S2CID 215775389.
- ↑ Jinsong, Huang (2020). "Resolving spatial and energetic distributions of trap states in metal halide perovskite solar cells". Science. 367 (6484): 1352–1358. arXiv:2008.06789. Bibcode:2020Sci...367.1352N. doi:10.1126/science.aba0893. PMID 32193323. S2CID 213193915.
- ↑ Nazeeruddin, Mohammad Khaja (2020). "Gradient band structure: high performance perovskite solar cells using poly(bisphenol A anhydride-co-1,3-phenylenediamine)". Journal of Materials Chemistry A. 8 (17113).
- ↑ Yang, Yang (2019). "Constructive molecular configurations forsurface-defect passivation of perovskite photovoltaics". Science. 366 (6472): 1509–1513. Bibcode:2019Sci...366.1509W. doi:10.1126/science.aay9698. OSTI 1574274. PMID 31857483. S2CID 209424432.
- ↑ Snaith, Henry J. (2014). "Enhanced Photoluminescence and Solar Cell Performance via Lewis Base Passivation of Organic-Inorganic Lead Halide Perovskites". ACS Nano. 8 (10): 9815–9821. doi:10.1021/nn5036476. PMID 25171692.
- ↑ Zhou, Zhongmin (2021). "Reducing Defects Density and Enhancing Hole Extraction for Efficient Perovskite Solar Cells Enabled by π-Pb2+ Interactions". Angewandte Chemie International Edition. 60 (32): 17356–17361. doi:10.1002/anie.202102096. PMID 34081389. S2CID 235321221.
- ↑ Fang, Junfeng (2018). "In-situ cross-linking strategy for efficient and operationally stable methylammoniun lead iodide solar cells". Nature Communications. 9 (1): 3806. Bibcode:2018NatCo...9.3806L. doi:10.1038/s41467-018-06204-2. PMC 6143610. PMID 30228277.
Further reading
- ASTM (1 March 2010), ASTM A967: Standard specification for chemical passivation treatments for stainless steel parts (Rev 05e2 ed.), doi:10.1520/A0967-05E02. The most common commercial spec for passivation of stainless steel parts. Used in various industries; latest revision is active for new designs; legacy designs may still require older revisions or older standards, if the engineering has not been revisited.
{{citation}}
: CS1 maint: postscript (link) - SAE (8 July 2011), AMS 2700: Passivation of corrosion resistant steels. (Rev D ed.). AMS specs are frequently used in the aerospace industry, and are sometimes stricter than other standards. Latest revision is active for new designs; legacy designs may still require older revisions or older standards, if the engineering has not been revisited.
{{citation}}
: CS1 maint: postscript (link) - SAE (16 February 2005), AMS QQ-P-35: Passivation treatments for corrosion-resistant steel (Rev A ed.). AMS-QQ-P-35 superseded U.S. federal spec QQ-P-35 on 4 April 1997. AMS-QQ-P-35 itself was canceled and superseded in February 2005 by AMS 2700.
{{citation}}
: CS1 maint: postscript (link) - U.S. government, QQ-P-35: Federal specification: Passivation treatments for corrosion-resistant steel (Rev C ed.). U.S. federal spec QQ-P-35 was superseded by AMS-QQ-P-35 on 4 April 1997 as part of the changeover instituted by the Perry memo. Both are now outdated; they are inactive for new designs, but legacy designs may still require their use, if the engineering has not been revisited.
{{citation}}
: CS1 maint: postscript (link) - Chromate conversion coating (chemical film) per MIL-DTL-5541F for aluminium and aluminium alloy parts
- A standard overview on black oxide coatings is provided in MIL-HDBK-205, Phosphate & Black Oxide Coating of Ferrous Metals. Many of the specifics of Black Oxide coatings may be found in MIL-DTL-13924 (formerly MIL-C-13924). This Mil-Spec document additionally identifies various classes of Black Oxide coatings, for use in a variety of purposes for protecting ferrous metals against rust.
- Budinski, Kenneth G. (1988), Surface Engineering for Wear Resistance, Englewood Cliffs, New Jersey: Prentice Hall, p. 48.
- Brimi, Marjorie A. (1965), Electrofinishing, New York, New York: American Elsevier Publishing Company, Inc, pp. 62–63.
- Bockris, John O'M.; Reddy, Amulya K. N. (1977), Modern Electrochemistry: An Introduction to an Interdisciplinary Area, vol. 2, Plenum Press, ISBN 0-306-25002-0.
- Passivisation : Debate over Paintability http://www.coilworld.com/5-6_12/rlw3.htm Archived 4 March 2016 at the Wayback Machine